Carbon Capture “Miracle Material” Breakthrough: MOF-525 Can Capture and Convert CO2 Into Useful Chemicals
Researchers have made a significant advancement in the practical application of a novel material known as MOF-525, a member of the metal-organic frameworks family, which shows great promise in carbon capture and conversion technologies. The team has developed a scalable manufacturing process using solution shearing techniques that allows MOF-525 to be applied in large areas, thus enhancing its effectiveness in capturing and converting carbon dioxide into commercially valuable chemicals. Credit: SciTechDaily.comResearchers at the University of Virginia have developed a scalable method for fabricating MOF-525, a material that can effectively capture and convert carbon dioxide into useful chemicals. This breakthrough offers a practical solution for large-scale applications in carbon capture and conversion, presenting significant environmental and energy benefits.Scientists have figured out how to take a miracle material, one capable of extracting value from captured carbon dioxide, and do what no one else has: make it practical to fabricate for large-scale application. Researchers at the University of Virginia School of Engineering and Applied Science conducted the study, which was published in ACS Applied Materials & Interfaces.The breakthrough from chemical engineering assistant professor Gaurav “Gino” Giri’s lab group has implications for the cleanup of the greenhouse gas, a major contributor to the climate change dilemma. It could also help solve the world’s energy needs. The Power of MOF-525The substance, called MOF-525, is in a class of materials called metal-organic frameworks.“If you can make these MOFs cover large areas, then new applications become possible, like making a membrane for carbon capture and electrocatalytic conversion all in one system,” Giri said.Electrocatalytic conversion creates a bridge from renewable energy sources to direct chemical synthesis, taking the burning of carbon dioxide-producing fossil fuels out of the equation.Assistant professor of chemical engineering Gaurav Giri. Credit: Tom CogillAdvancing Carbon Capture SolutionsWhat gives MOFs superpowers is their ultra-porous, crystalline structures — 3D networks of minute nanoscale cavities that create vast internal surface area and act like a sponge — that can be designed to trap all sorts of chemical compounds.Giri’s group reasoned that starting with an inherently scalable synthesis technique — solution shearing — would better their odds. They had already had success shearing simpler MOFs.In Giri’s process, the MOF’s components are mixed in a solution, and then spread across a substrate with the shearing blade. As the solution evaporates, chemical linkages form the MOF as a thin film on the substrate. Applying MOF-525 in this way produces an all-in-one membrane for carbon trapping and conversion.Scaling Up for Greater Impact“The bigger the membrane, the more surface area you have for the reaction, and the more product you could get,” said Prince Verma, a December 2023 Ph.D. graduate from Giri’s lab. “With this process, you can increase the shearing blade width to whatever size you need.”The team targeted CO2 conversion to demonstrate their solution shearing approach because carbon capture is widely used to reduce industrial emissions or to remove it from the atmosphere — but at a cost to operators with minimal return on the investment: Carbon dioxide has little commercial value and most often winds up stored indefinitely underground.However, with minimal energy input, using electricity to catalyze a reaction, MOF-525 can take away an oxygen atom to make carbon monoxide — a chemical that is valuable for manufacturing fuels, pharmaceuticals, and other products.UVA’s Commitment to Green EnergyThe process of accelerating reactions through catalysis, especially electrocatalysis, which consumes less energy than reactions driven by heat or pressure, is essential to a green-energy future — so much so that UVA invested $60 million in catalysis study as part of UVA’s Grand Challenges Investments.For that expertise, Giri collaborated with UVA associate professor of chemistry Charles W. Machan.“The materials from Gino’s lab help us understand how to enable new, scalable technologies for capture and conversion, which we’re going to need to address the environmental challenges posed by current carbon dioxide concentrations in the atmosphere and rate of emissions,” Machan said. The researchers published their findings in the American Chemical Society journal Applied Materials and Interfaces.Reference: “Solution Shearing of Zirconium (Zr)-Based Metal–Organic Frameworks NU-901 and MOF-525 Thin Films for Electrocatalytic Reduction Applications” by Prince K. Verma, Connor A. Koellner, Hailey Hall, Meagan R. Phister, Kevin H. Stone, Asa W. Nichols, Ankit Dhakal, Earl Ashcraft, Charles W. Machan and Gaurav Giri, 13 November 2023, ACS Applied Materials & Interfaces.DOI: 10.1021/acsami.3c12011Also contributing to the work were Connor A. Koellner, Hailey Hall, Meagan R. Phister, Kevin H. Stone, Asa W. Nichols, Ankit Dhakal and Earl Ashcraft.The research was supported by the UVA Environmental Institute; the U.S. Department of Energy, Office of Science, Office of Basic Energy Sciences, Catalysis Science Program; the Nanoscale Materials Characterization Facility at UVA; and the Stanford Synchrotron Radiation Lightsource, SLAC National Accelerator Laboratory.
Researchers at the University of Virginia have developed a scalable method for fabricating MOF-525, a material that can effectively capture and convert carbon dioxide into...
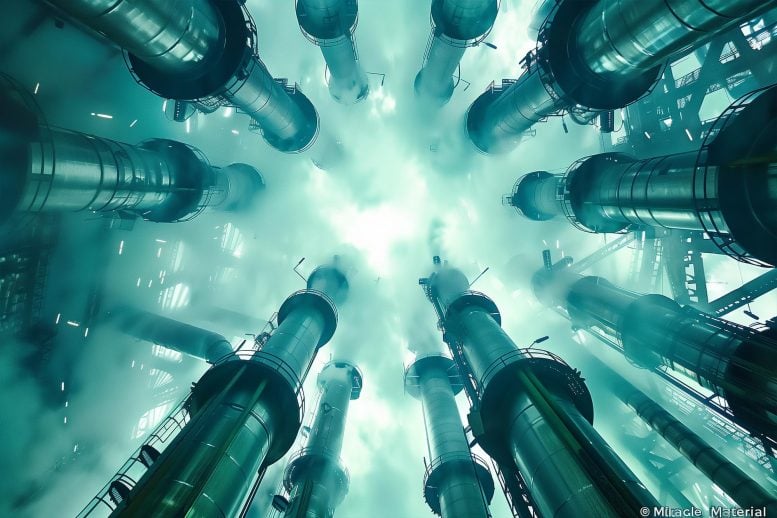
Researchers have made a significant advancement in the practical application of a novel material known as MOF-525, a member of the metal-organic frameworks family, which shows great promise in carbon capture and conversion technologies. The team has developed a scalable manufacturing process using solution shearing techniques that allows MOF-525 to be applied in large areas, thus enhancing its effectiveness in capturing and converting carbon dioxide into commercially valuable chemicals. Credit: SciTechDaily.com
Researchers at the University of Virginia have developed a scalable method for fabricating MOF-525, a material that can effectively capture and convert carbon dioxide into useful chemicals. This breakthrough offers a practical solution for large-scale applications in carbon capture and conversion, presenting significant environmental and energy benefits.
Scientists have figured out how to take a miracle material, one capable of extracting value from captured carbon dioxide, and do what no one else has: make it practical to fabricate for large-scale application. Researchers at the University of Virginia School of Engineering and Applied Science conducted the study, which was published in ACS Applied Materials & Interfaces.
The breakthrough from chemical engineering assistant professor Gaurav “Gino” Giri’s lab group has implications for the cleanup of the greenhouse gas, a major contributor to the climate change dilemma. It could also help solve the world’s energy needs.
The Power of MOF-525
The substance, called MOF-525, is in a class of materials called metal-organic frameworks.
“If you can make these MOFs cover large areas, then new applications become possible, like making a membrane for carbon capture and electrocatalytic conversion all in one system,” Giri said.
Electrocatalytic conversion creates a bridge from renewable energy sources to direct chemical synthesis, taking the burning of carbon dioxide-producing fossil fuels out of the equation.
Advancing Carbon Capture Solutions
What gives MOFs superpowers is their ultra-porous, crystalline structures — 3D networks of minute nanoscale cavities that create vast internal surface area and act like a sponge — that can be designed to trap all sorts of chemical compounds.
Giri’s group reasoned that starting with an inherently scalable synthesis technique — solution shearing — would better their odds. They had already had success shearing simpler MOFs.
In Giri’s process, the MOF’s components are mixed in a solution, and then spread across a substrate with the shearing blade. As the solution evaporates, chemical linkages form the MOF as a thin film on the substrate. Applying MOF-525 in this way produces an all-in-one membrane for carbon trapping and conversion.
Scaling Up for Greater Impact
“The bigger the membrane, the more surface area you have for the reaction, and the more product you could get,” said Prince Verma, a December 2023 Ph.D. graduate from Giri’s lab. “With this process, you can increase the shearing blade width to whatever size you need.”
The team targeted CO2 conversion to demonstrate their solution shearing approach because carbon capture is widely used to reduce industrial emissions or to remove it from the atmosphere — but at a cost to operators with minimal return on the investment: Carbon dioxide has little commercial value and most often winds up stored indefinitely underground.
However, with minimal energy input, using electricity to catalyze a reaction, MOF-525 can take away an oxygen atom to make carbon monoxide — a chemical that is valuable for manufacturing fuels, pharmaceuticals, and other products.
UVA’s Commitment to Green Energy
The process of accelerating reactions through catalysis, especially electrocatalysis, which consumes less energy than reactions driven by heat or pressure, is essential to a green-energy future — so much so that UVA invested $60 million in catalysis study as part of UVA’s Grand Challenges Investments.
For that expertise, Giri collaborated with UVA associate professor of chemistry Charles W. Machan.
“The materials from Gino’s lab help us understand how to enable new, scalable technologies for capture and conversion, which we’re going to need to address the environmental challenges posed by current carbon dioxide concentrations in the atmosphere and rate of emissions,” Machan said.
The researchers published their findings in the American Chemical Society journal Applied Materials and Interfaces.
Reference: “Solution Shearing of Zirconium (Zr)-Based Metal–Organic Frameworks NU-901 and MOF-525 Thin Films for Electrocatalytic Reduction Applications” by Prince K. Verma, Connor A. Koellner, Hailey Hall, Meagan R. Phister, Kevin H. Stone, Asa W. Nichols, Ankit Dhakal, Earl Ashcraft, Charles W. Machan and Gaurav Giri, 13 November 2023, ACS Applied Materials & Interfaces.
DOI: 10.1021/acsami.3c12011
Also contributing to the work were Connor A. Koellner, Hailey Hall, Meagan R. Phister, Kevin H. Stone, Asa W. Nichols, Ankit Dhakal and Earl Ashcraft.
The research was supported by the UVA Environmental Institute; the U.S. Department of Energy, Office of Science, Office of Basic Energy Sciences, Catalysis Science Program; the Nanoscale Materials Characterization Facility at UVA; and the Stanford Synchrotron Radiation Lightsource, SLAC National Accelerator Laboratory.